[ad_1]
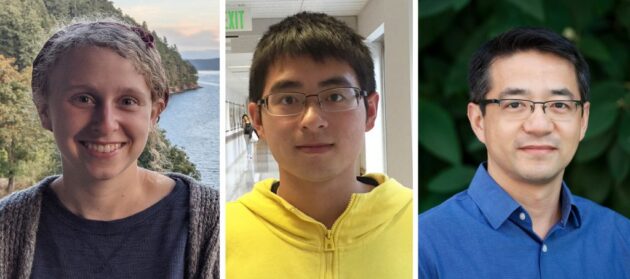
Most of us don’t think of atoms as having their own unique vibrations, but they do. In fact, it’s a feature so fundamental to nature’s building blocks that a team of University of Washington researchers recently observed and used this phenomenon in their research study. By studying the light atoms emitted when stimulated by a laser, they were able to detect vibrations sometimes referred to as atomic “breathing.”
The result is a breakthrough that may one day allow us to build better tools for many kinds of quantum technologies.
Led by Mo Li, a professor of photonics and nano devices in both the UW Department of Electrical and Computer Engineering and the UW Physics Department, the researchers set out to build a better quantum emitter, or QE, one that could be incorporated into optical circuits.
QEs are an essential part of the quantum technology toolkit in that they provide a way to generate individual quantum particles that can be used as qubits. Analogous to bits of information in everyday computing, qubits are used in quantum computing to perform calculations far beyond what can be achieved with classical computers. Typically, a qubit is built from an electron or a photon because of these particle’s unique quantum properties.
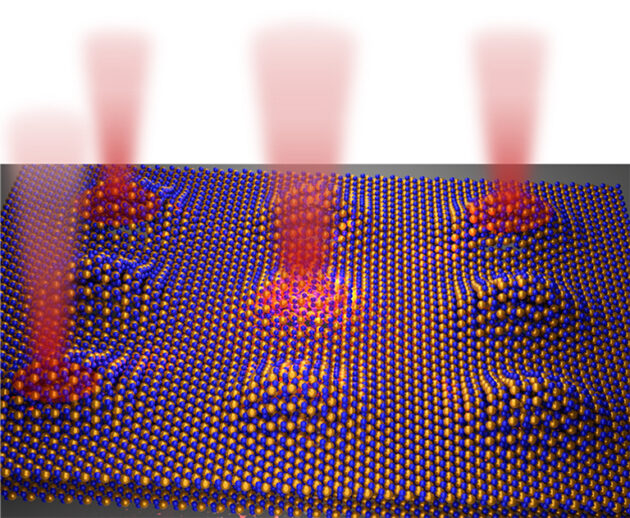
“This is a new, atomic-scale platform, using what the scientific community calls ‘optomechanics,’ in which light and mechanical motions are intrinsically coupled together,” said Li. “It provides a new type of involved quantum effect that can be utilized to control single photons running through integrated optical circuits for many applications.”
To build their QE, the team began with tungsten diselenide, a molecule composed of tungsten and selenium. This was formed into the thinnest of sheets, each only a single atom thick. Two of these sheets were then layered one atop the other and placed over a series of nanopillars, a mere 200 nanometers wide.
This placement on the nanopillars caused the sheets to deform at the point of contact, resulting in a series of regularly spaced quantum dots. Quantum dots are semiconductor particles a few nanometers in size, having unique optical and electronic properties and are a common method of building QEs for quantum applications. Because of the deformation caused by the nanopillars, these are more specifically referred to as “strain-induced quantum dots.”
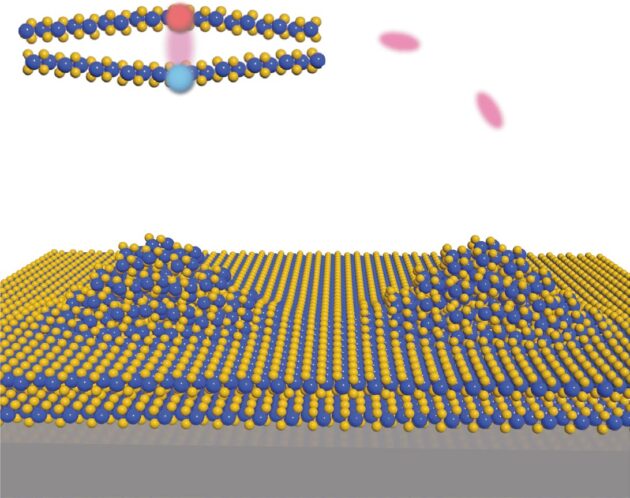
By applying a precise pulse of laser light to one of the quantum dots, an electron is knocked away from the tungsten diselenide atom’s nucleus. This briefly creates a quasiparticle known as an exciton. This exciton is composed of the negatively charged electron and the corresponding positively charged hole in the opposite sheet. Because they are strongly bound, the electron quickly returns to the atom. When it does this, it releases a single photon encoded with very specific quantum information.
“To feasibly have a quantum network, we need to have ways of reliably creating, operating on, storing and transmitting qubits,” said Adina Ripin, a lead author of the paper, member of the Mo Li Group, and a doctoral student in the physics department. “Photons are a natural choice for transmitting this quantum information because optical fibers enable us to transport photons long distances at high speeds, with low losses of energy or information.”
This approach resulted in producing very consistent, high-quality photons that could potentially be used as qubits. By itself, this would make the project a success. However, certain details soon became apparent in the data, meriting a deeper look.
The researchers found that a quasiparticle called a phonon was also being produced in the process of creating each photon. Phonons are an optomechanical phenomenon based on the vibration between atoms and they occur in all matter. Phonons can be thought of as acoustic analogs to photons, with their own quantum waveforms. Though we can’t directly see or hear this, Li says the vibrations can be visualized as the “breath between atoms.”
In this study, the phonons were generated by the vibration between the two atom-thin layers of tungsten diselenide, which acted like tiny drumheads vibrating relative to each other. The UW team found these phonons were tightly correlated to the photon that was being generated.
“You can think of phonons in terms of a little spring attached to the layers,” Li said. “This spring is vibrating, so it directly changes how the electron and the hole can recombine. Because of this, the photon that’s emitted changes as well.”
Previously, phonons had never been observed in this type of single photon emitter system. Moreover, when analyzing the spectrum of the light emitted, the team found equally spaced peaks representing the phonon’s different quantum energy levels. Expert analysis by Ting Cao, a quantum theorist and an assistant professor in materials science and engineering, revealed that every single photon emitted by an exciton was coupled with one, two, three or more phonons.
“A phonon is the natural quantum vibration of the tungsten diselenide material, and it has the effect of vertically stretching the exciton electron-hole pair sitting in the two layers,” Li continued. “This has a remarkably strong effect on the optical properties of the photon emitted by the exciton that has never been reported before.”
The team was further able to tune the phonon-exciton-photon interaction by applying electrical voltage across the materials. By varying the voltage, they found they could alter the interaction energy of the associated phonons and emitted photons. This was controllable in ways relevant to encoding specific quantum information into a single photon.
“I find it fascinating that we were able to observe a new kind of hybrid quantum platform,” said Ruoming Peng, also a lead author of the paper, who graduated with his doctoral degree from UW ECE in 2022. “By studying the way phonons interact with quantum emitters, we discovered a whole new realm of possibilities for controlling and manipulating quantum states. This could lead to even more exciting discoveries in the future.”
Li and his team want to extend their system further, controlling multiple emitters and their associated phonon states. Doing this would allow the quantum emitters to “talk” to each other, building the basis for new kinds of quantum circuitry. Future applications for these approaches include quantum computing, quantum communications and quantum sensing.
The UW team includes Adina Ripin, Ruoming Peng, Xiaowei Zhang, Srivatsa Chakravarthi, Minhao He, Xiaodong Xu, Kai-Mei Fu, Ting Cao, and Mo Li. The research is supported by the National Science Foundation. Their research paper, “Tunable phononic coupling in excitonic quantum emitters” was recently published in the journal Nature Nanotechnology.
[ad_2]
Source link